Company using microbes to make spider silk garners $123,000,000 in venture capital.
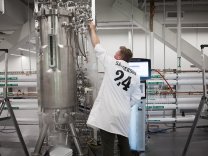
This is a story reported on the Tech Crunch site, about Bolt Threads. The proposed uses seem to center on wearable textiles (vs. engineering textiles). Why spider silk? It’s touted to be stronger than other polymers – stronger than Teflon, in the Tech Crunch page. That’s a mistake, since they meant Kevlar, the amazing polymer used in bulletproof vests. Spider silk is also claimed to be stronger than steel. Consider these points:
- “Strength” is one of many properties of a structural material (yes, textiles are structures of a special sort); stiffness and toughness are equally important.
- These properties commonly trade off against each other, and in any application of a material – polymer, steel, carbon fiber, etc. – there are choices to make. Brick is a strong material but it’s very un-tough, as are ceramics in general. Steels are strong but the strongest steels aren’t the toughest (meaning taking the most energy to break). I have a quick summary of properties below.
- Many structural materials have properties that vary with the environment. The steel in WW II Liberty ships became brittle at cold North Atlantic Ocean conditions, and they sometimes broke apart under wave stresses.
- Spider silk is notorious for its properties depending strongly on humidity and temperature.
Another property of note is resilience. When stressed (stretched along its length, for example, some spider silk stays stretched. Not so good for a tie. Of course, silkworm silk is good here (doesn’t stretch, is stiff), and some types of spider silk are resilient, and stiff, like silkworm silk. Pick the right type of spider silk, and perhaps blend several types.
- As many investigators have noted, spider silks vary; some classifications put as many as 27 types in play. Bolt Thread likely will need to make a number of different silks. Look at orb-weaving spiders, the common web-makers. They use strong and resilient silk for the main radial lines and nonresilient, sticky silk for the cross lines. The strong lines keep the web intact, but they would just kick an insect back out rather than trap it. The sticky lines do the trapping.
-
- One use I saw touted the use of spider silk instead of steel cables on aircraft carriers for arresting the landing of an aircraft. Two scenarios seem to have be implied.
- One is that the trapping type of silk, or flagelliform silk, be used, as if catching a very big fly. As Stephen Vogel notes in his oh-so-readable book, Cats’ Paws and Catapults, spider silk is nonresilient; the energy it would absorb would go into making so much heat that the net would melt. So, that won’t work.
- The other is using spider silk as the arresting cables, the ones that the aircraft’s tailhook engages, or perhaps the balancing cables that take the shock of stopping a landing aircraft by paying out from a drum with hydraulic damping, like big car shock absorbers. There, the tough and strong silk might be used. A variant arresting method, for aircraft missing a tailhook, is to put out a big net instead of the arresting cable. OK, but why go to spider silk, which is likely harder to maintain and in a function where weight is not critical?
- Spider silk really isn’t stronger than Kevlar. Both (some) spider silk (drag lines) and Kevlar are stronger than steel, and notably better than stell per unit weight, but Kevlar is stronger than spider silk by a factor of 1.5 to 7. Nothing is better for strength.
Here’s a rundown of strength, stiffness, toughness, resilience, ductility, and some shape dependence of material failure. We can conclude at the end that spider silk has a mix of properties that suit it to certain uses, choosing it over Kevlar (or steel, or putty, or ….), but not to other uses. Using it for high-priced silk ties is an affectation for the rich. I’ll wait to hear what real uses are in line.
We can look at a graph of the extent to which a material deforms (its fractional change in length) against the stress put on it (the force over an area). Such a plot is useful for materials that have the same properties in any direction, or isotropic materials, though one can use it for materials whose properties vary by direction, if one specifies the direction. (There are also differences in stresses applied in one direction, as extension or compression, and shear stresses applied nonuniformly, as in trying to stretch a material into a distorted rectangle or parallelogram.)
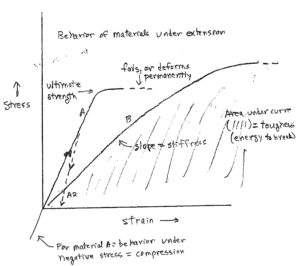
Here’s a simple comparison of two materials, well, OK, three. Material A deforms a lot less than does material B, under the same stress. Or, you can say that material A takes a lot more stress to deform it by a given amount. It has greater stiffness. We can take the slope of stress vs. strain at any point and call it stiffness. For many materials, stiffness is pretty constant over a range of stress levels – e.g., steel.
Strength is the amount of stress needed to make the material break apart. Material A isn’t as good as B.
Toughness is the total energy needed to make a material fail. It’s the area under the curve from zero stress to failure. Again, B is better.
Resilience is the ability to return to the original shape when stress is relieved. We tend to like that. Spring steel needs to be resilient. Putty is non-resilient, and we like that for keeping it in place once we apply it. I drew a line on the side of a related but distinct material, A2, indicating what strain remains when the stress goes down. It ends with a non-zero strain when stress is completely removed.
Material A2 is not fully resilient; it might be resilient, however, from its new, once-stretched state, under later stresses. If so, we can call the material ductile, able to be deformed and take on a new, resilient state. It’s what we do in forming metals with dies by stamping, pulling through wire dies, forging, etc.
We can also call resilience elasticity. The opposite of it is plasticity – undergoing plastic deformation.
I’ve attached another graph with an explicit comparison of spider silk to Kevlar
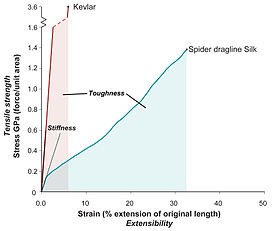
OK, spider silk is tougher than Kevlar if not stronger and not stiffer (of course, we knew it wasn’t stiffer!). Its resilience depends on how far you stretch it – probably not as resilient as Kevlar, but close.